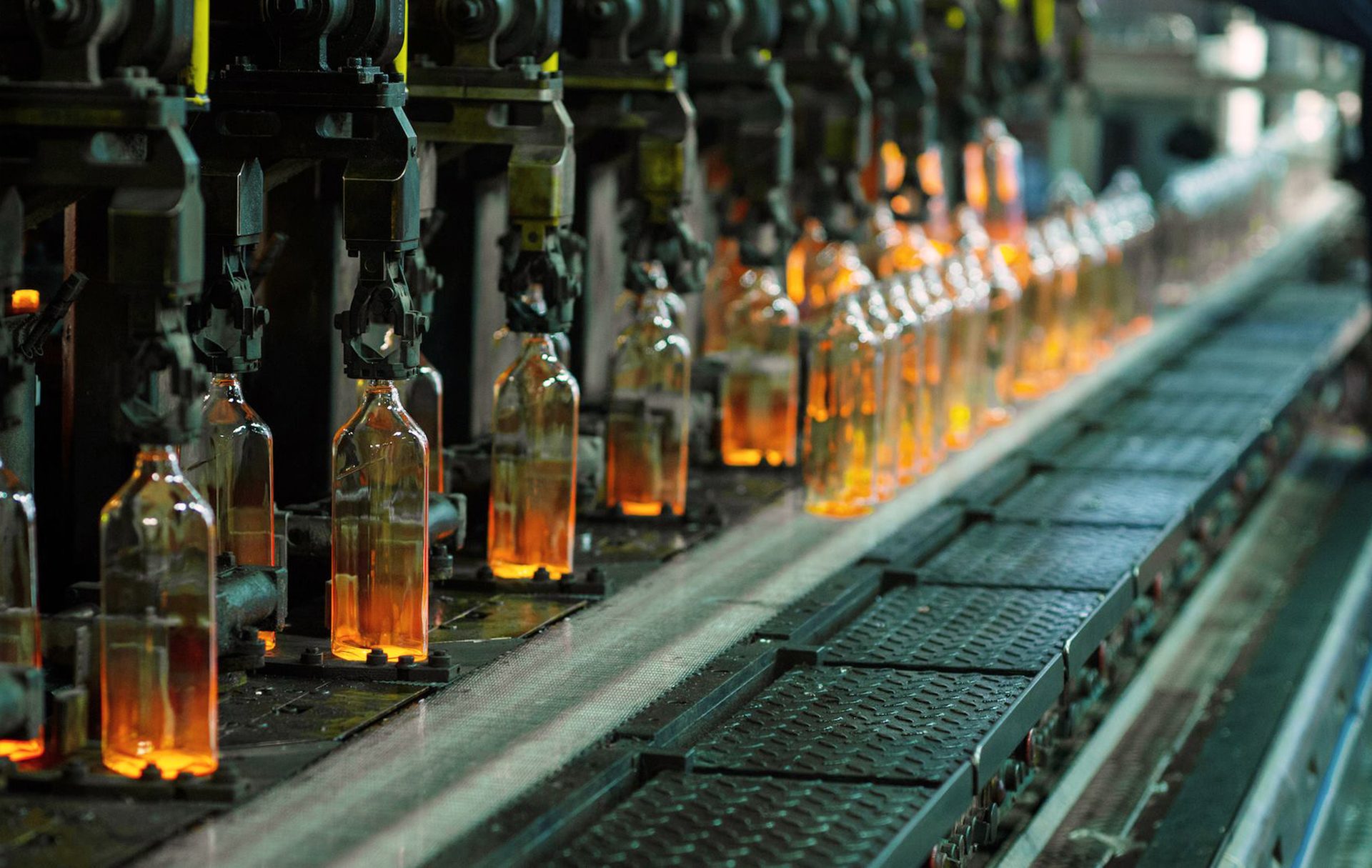
The future for the glass industry is all-electric
By René Meuleman
Meuleman is the solutions and technology lead for green glass at Paris-based Schneider Electric. This article was originally presented at the 14th International Seminar on Furnace Design at Vsetin, Czech Republic, in 2017. View the original article here. Reproduced with permission.
Glass melting has been carried out for nearly 6,000 years, and, for most of that time, wood was used as the energy source. It was only comparatively recently, around 1880, that the industry began to use fossil fuels like oil and natural gas. At this time, the regenerator had already been invented to improve the efficiency of steel blast furnaces, and this invention was soon adapted by the glass industry on the early port furnaces, very similar to how we know them today. During those thousands of years of glassmaking, less than 150 years’ worth of fossil fuels were used, and it is possible that they will not be around for another 150 years.
Although new fossil fuel resources have recently become available, the world has at last begun to understand that burning them results in unavoidable carbon emissions, and therefore this method must come to an end. Glass melting still needs to continue at this point in time because we have not yet discovered a viable replacement material. It is therefore likely that glass will be around for many centuries to come and that the inevitable future for a carbon efficient glass industry will be “all-electric.”
ADVERTISEMENT
History
With no disrespect to past furnace design developments and the great achievements that have been made, they are mostly still based on original technology. Traditional side and end port furnaces are proven technology that has been developed and tweaked to a level of efficiency, low emissions, and life expectancy that simply cannot be improved any further. Since the efficiency level came down to 2.4 MWh/ton in around 1990, no big improvements have been achieved. Consequently, further CO2 and NOx emission reductions slowed to halt as well.
Oxy-fuel firing, batch preheating, waste heat recovery, submerged burners, etc. are great advances, but the bottom line remains the same: they all increase the complexity of the melting system and CAPEX, do not avoid CO2 emissions, and in most cases cannot reduce NOx emissions any further. The use of fossil fuels has become the fundamental problem and technology cannot overcome these issues sufficiently.
The fuzziness of politics
Just as with many other raw materials, as soon as we start believing that resources are coming to an end, we find new ones. That is also applicable for fossil fuels. So why should we even start considering diverting from fossil fuels? Science has proven that CO2 emissions are related to global warming, which will likely lead to serious environmental issues for humanity. Legislation, customers, and common sense will force the industry to step away from fossil fuel firing sooner or later.
By 2050, the EU aims to cut greenhouse gas emissions to 80% below 1990 levels. Milestones to achieve this goal are 40% emissions cuts by 2030 and 60% by 2040. All sectors need to contribute. One famous Dutch beer brewer is putting a lot of effort into reducing its carbon footprint and estimates that 53% of this footprint is related to its packaging material. The pressure to reduce emissions comes from many sides. No matter which side we agree or disagree with, it will impact how glass is melted in the future.
Technological evidence
Most glass melting furnace technology goes back 100 years or more. Over the years, different developments have led to huge energy efficiency and emission improvements, and many furnace suppliers are still working on enhancements, forced by the fact that fossil fuel energy remains cheap. However, that will change, and probably much faster than many of us expect.
As previously mentioned, most of those improvements implicate a more complex technology that results in additional maintenance and CAPEX, the use of nonenvironmentally friendly chemicals, and limitations to equipment lifespan. Most glass smelters perceive their melting process as complex enough and are not keen on modifying it further. They want to focus on their core business, without the issues of managing and maintaining complex industrial installations requiring high numbers of technical personnel. Keeping the system simple has been a key argument for many decades.
Now that the world around us seems to be changing rapidly, our efforts to extend the lifetime of furnaces up to 15 years or more is working against us. In fact, most glass manufacturers only have one opportunity every 10 to 15 years to introduce a new innovative melting process, so it is not surprising that having to live with that decision for the next 15 years makes them extremely risk averse. Who can blame them? It reminds me of a comment made by one of our customers: “In God we trust, but here you have to come with facts.” Technological research and development needs to provide evidence of improvements, otherwise politics forces us to rely on expectations.
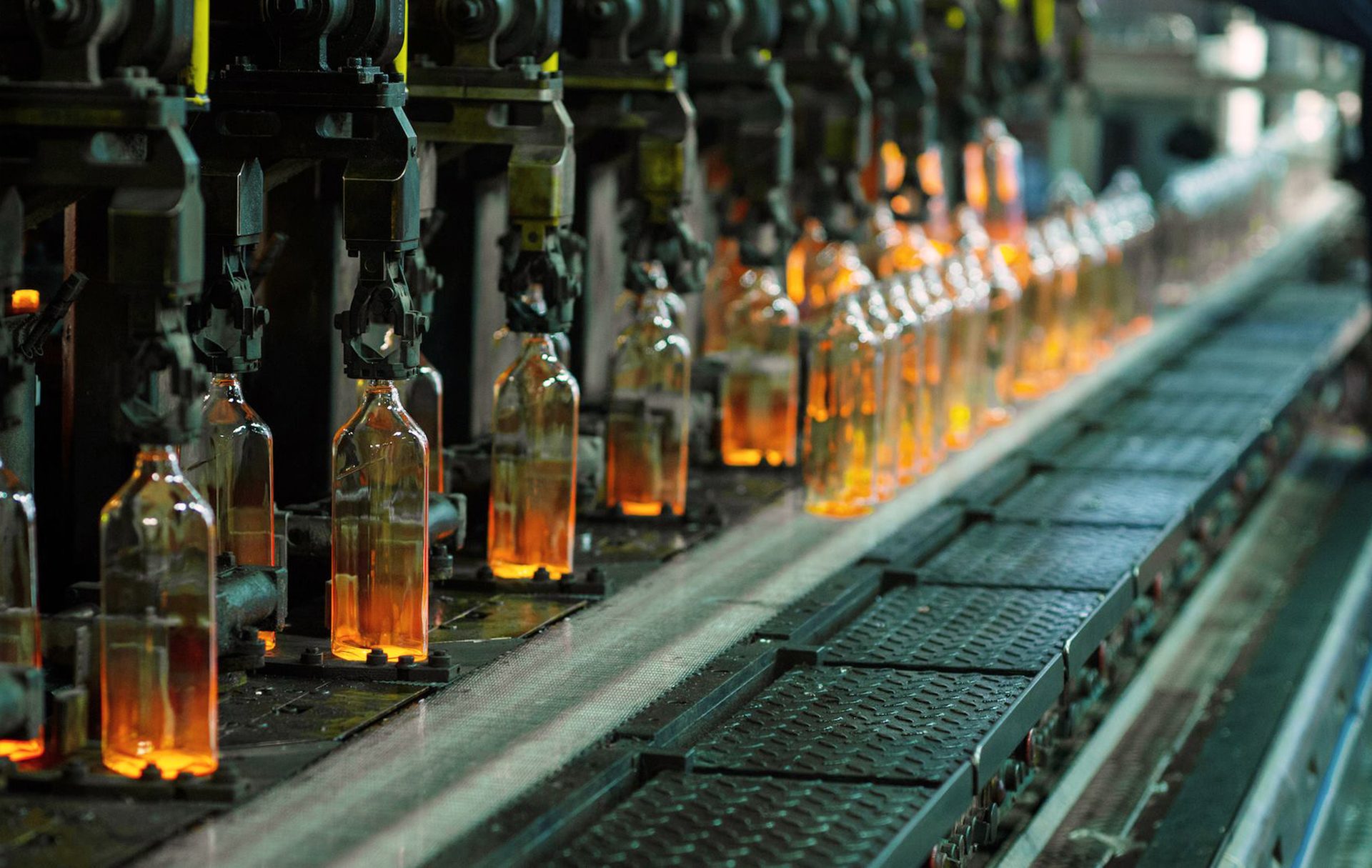
Credit: Ardagh Group
Electrical heating
Electrically heated furnace technology is almost as old as regenerative furnace technology. In fact, the first furnace patent on electrical melting was issued to Sauvageon in France, in 1907. A first successful cold top furnace ran in Norway from 1920 to 1925 using carbon electrodes. Cornelius in Sweden had operating furnaces as early as 1925, producing amber and green glass.
In 1952, the industry started to use molybdenum electrodes, and around 1975 high current SCR’s (thyristors) became available, leading to the principle of solid-state furnace boosting systems we know today. Most modern traditional container, fiber, and float furnaces are now equipped with electrical furnace boosting, contributing 10% to 50% of the melting power.
The efficiency of all-electric melting
Even in the early days, all-electric melting efficiency at 4.4 GJ/ton (1.3 MWh/ton) was already close to today’s most efficient fossil fuel fired furnaces at 4 GJ/ton (1.1 MWh/ton). Since the introduction of all-electric furnaces, huge efficiency improvements have been achieved, reducing energy usage levels to 2.8 GJ/ton (0.78 MWh/ton) (20% cullet) or less. The power consumption is not likely to go below 2.6 GJ/ton (0.72 MWh/ton). Most of the electrical power ends up in the melting process anyway, and only relatively low energy losses come from transformers, busbar, and control efficiency. Compared to traditional fossil fuel heating at 4 GJ/ton (1.1 MWh/ton), energy use is around 35% less.
An electrical furnace is naturally easy to control and maintain, but it is important to consider the engineering of the electrical system alongside the furnace design. Like a burner system for a traditional furnace, the electrical system is not a sub system but should be part of the total design and needs to be fully integrated. Bringing steelwork, refractory, cables, busbars, electrodes, transformers, and control together in one design is essential for the efficiency success of the whole system.
Advantages of all-electric melting
Compared to high-efficiency, fossil-fuel-fired smelter systems, all-electric furnaces are sophisticated but very straightforward in design. Regenerators or burner skids are not required, and expensive high-temperature crowns are not necessary. Higher pull rates can be achieved without any problems. No combustion related CO2, thermal NOx or SOx emissions are released. Potentially less evaporation of volatile and expensive raw materials, such as boron and lithium, will occur, which makes exhaust filtering much easier. Also, the carryover problem will almost vanish. Smaller furnaces could be considered. For example, one furnace that feeds one forehearth which feeds one IS machine might become a new concept for bottle manufacturing.
Disadvantages of all-electric melting
Although all-electric furnace concepts are very simple in principle, there are some implications to consider when changing over to this technology. At room temperature, glass or glass compositions are electrical insulators. To start the electrical heating process, it needs to run through a preheating sequence similar to the method used in container and float furnaces.
An all-electric furnace also needs a stable, reliable power grid, and due to different melting and fining behaviors, the glass composition needs to be changed. Electrical tariffs need to come down in price, and in order to lower the carbon footprint, electricity would need to come from renewables instead of coal-fired power plants.
Electrodes need to be maintained by advancing them in case wear leads to higher resistance. There are new methods to counter electrode wear, which would need to be investigated further. Another issue, especially for the container industry, might be how this kind of furnace would handle extremely high amounts of cullet, which may result in different ways cullet and batch are managed.
Flexibility is required
Electrical power tariffs are strongly related to availability, and the electrical energy market is changing rapidly. Suppliers and utilities subsidized by government grants are investing in wind, biomass, and solar power generation. Citizens also invest in solar panels instead of keeping their money at zero interest in banks. Buzzwords such as “smart grid,” “tariff tweaking,” “peak shaving,” and “frequency control” have become familiar terms, and it is recognized that money can be saved if our electrical energy consuming system becomes more flexible.
To lower the risk of total grid failure, some utilities offer money to be in control of huge industrial loads, to be able to temporarily switch them off when needed. More refined is the method of controlling the network’s frequency (dynamic fractional frequency reuse) by tweaking the power consumption of some massive power consumers. Basically, electrical power consumers are financially rewarded if they make part of their electrical power consuming system available for remote power control. Lower peak power demand can lead to lower tariffs. In that case, a dynamic load management system capable of controlling parts of the electrical system to ensure that agreed peak power levels are not exceeded will lower the overall cost of electrical energy.
A glass furnace, containing a huge amount of molten glass, can or should be able to accommodate the flexibility needed to profit from these rewards, grants, and lower electrical tariffs. Glass manufacturing, being part of the high energy consumer community and rapidly changing energy market, needs to look for furnace designs that better fit both today’s and tomorrow’s requirements. Sophisticated data analysis and (model-based) control strategies should help operators to calculate the available freedom of control, allowable melting energy fluctuations, allowable fossil-to-electric ratio fluctuations, and predict the impact on glass quality. The bottom line is that there is no escape from thinking “out of the box” and stepping away from tradition.