journal highlights
Jonathon Foreman
ACerS journals managing editor
Recent advances to all-solid-state lithium batteries
Global science and engineering initiatives have brought us ever closer to a world that does not rely on carbon-based power generation. However, the growth of sustainable energy harvesting along with the electrification of transportation have been limited by the lack of efficient and cost-effective energy storage solutions.
Lithium-based batteries are among the leading technologies for energy storage. In lithium batteries, metallic lithium in the anode is converted into lithium ions while simultaneously releasing electrons, which carry the energy to the connected device. The lithium ions travel through the electrolyte to the cathode where they are deposited via reaction with the cathode material and the electrons returning to the battery.
There are many challenges to engineering lithium batteries that maximize the positive interactions among these three layers while minimizing the performance-limiting interactions, as described in the paper “A review on ‘Growth mechanisms and optimization strategies for the interface state of solid-state lithium-ion-batteries’” by Liu et al.
For example, regarding the cathode, the most commonly used material is lithium cobalt oxide (LiCoO2). The challenges of cobalt-based cathodes include relatively low energy capacity (theoretically 300 mA·h/g), reactions with electrolytes, potential for thermal runaway, and limited supply.
Of materials being explored to replace cobalt, sulfur has potential for substantially improved cathode capacity, with theoretical values of more than 1,500 mA·h/g. But side reactions in lithium–sulfur batteries limit the availability of sulfur and lithium and lead to capacity fade. In the article “Particle size control of cathode components for high-performance all-solid-state lithium–sulfur batteries,” Fan et al. demonstrated high capacity (800–1,000 mA·h/g) with minimal fade when using a nanoscale lithium sulfide/nanoscale sulfide-based electrolyte as a composite cathode.
Turning attention to the electrolyte, current lithium-ion batteries employ flammable liquid organic or polymer electrolytes that lack resistance to dendrite formation. In contrast, emerging all-solid-state lithium batteries (ASSLBs) use solid ceramics as the electrolyte (Figure 1), which can resist dendrite formation and mitigate the risk of the battery catching fire.
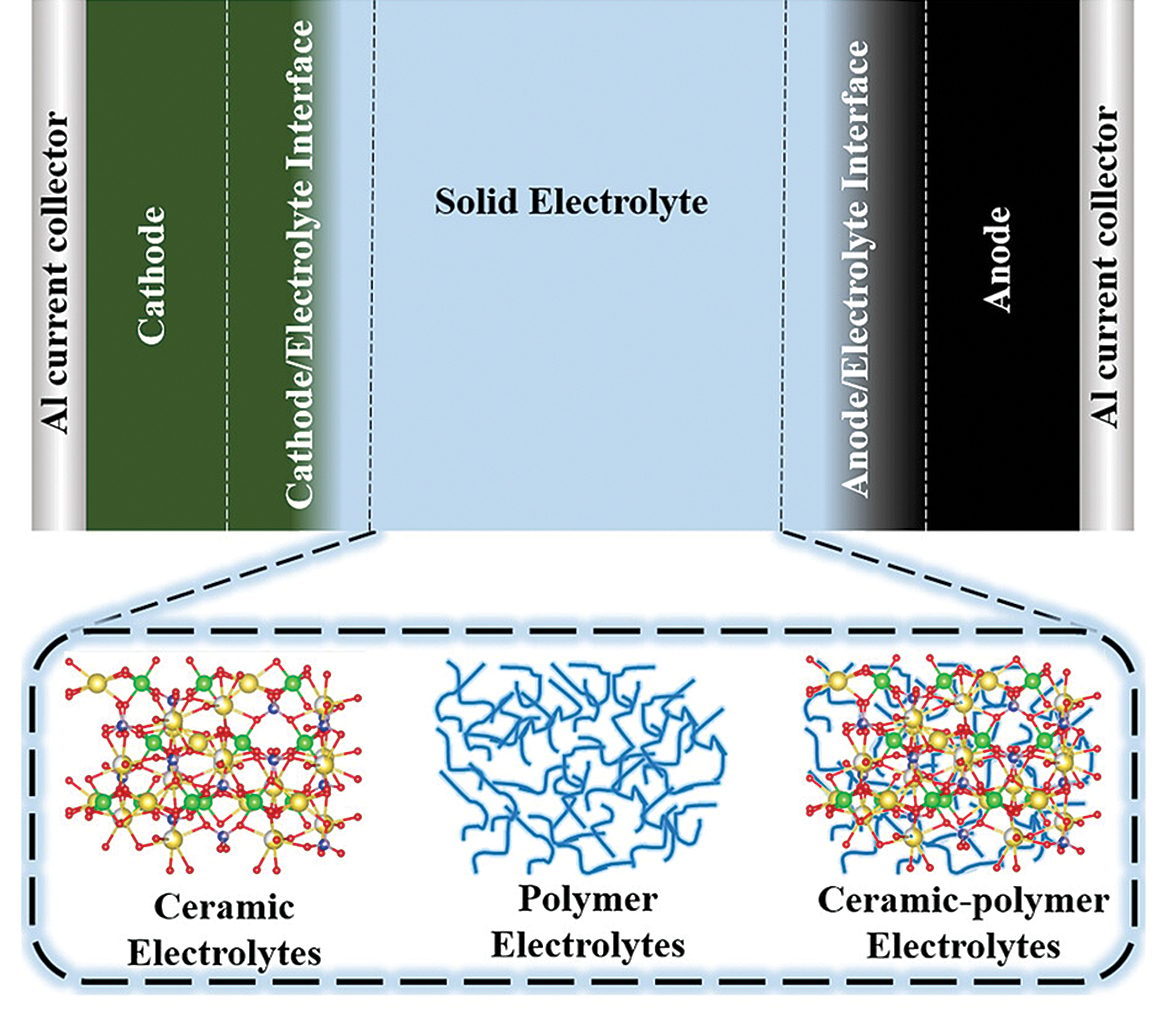
Figure 1. Schematic demonstration of a typical all-solid-state sodium battery comprising an anode and cathode integrated with various categories of solid electrolytes.
Credit: Vasudevan et al., International Journal of Applied Ceramic Technology
The three most common classes of ceramic electrolytes used in ASSLBs are lithium aluminum titanium phosphate (LATP), lanthanum zirconium oxide (LLZO), and lithium phosphorus sulfide (LiPS). LATP and LLZO tend to be brittle and are difficult to sinter. LiPS electrolytes are easier to sinter, but they are highly reactive with air and some cathode materials.
Many approaches are being explored for improving ceramic electrolytes. For example, in the articles “An amorphous superionic conductor Li3PS4-xLiBr with high conductivity and good air stability by halogen incorporation” by Wang et al. and “Nitrogen doped sulfide solid electrolytes with enhanced air stability and lithium metal compatibility” by Luan et al., they doped LiPS with bromine and nitrogen, respectively, to improve chemical integrity.
In addition, substantial efforts are being made toward improving the anodes of lithium-ion batteries. The most common anode is graphite, which suffers from low capacity (372 mA·h/g) and slow lithium-ion diffusion. Silicon and SiOx as anode materials offer higher capacities. But these undergo dramatic (100–400%) volume changes during the charge–discharge cycle, which damages the anode–electrolyte interface.
Glass and glass-ceramic anodes provide one avenue for mitigating volume changes. For example, in the paper “Synthesis of passively prelithiated SiOx nanoparticles for Li-ion battery anode,” Kim et al. fabricated lithiated nanoscale SiOx anodes containing amorphous SiO and various crystalline phases. The anodes exhibited capacities on the order of 1,000 mA·h/g and 75% retention after 50 cycles.
Some ASSLBs have no anode at all. The so-called anode-less cells are assembled with pre-lithiated cathodes and metal current collectors instead of intercalated anodes. Upon charging, the lithium from the cathode plates directly onto the metal current collector. This method tends to minimize the probability of dendrite formation because no diffusion occurs at the anode.
In the paper “Effect of cell pressure on the electrochemical performance of all-solid-state lithium batteries with zero-excess Li metal anode,” Park et al. tested how pressure affects the performance of this type of cell. They found high pressures and/or spring-loading effectively accommodates cathode and anode volume changes with excellent capacity retention up to 97% over 50 cycles.
Get more information on the articles in the Topical Collection “Recent advances to all solid-state lithium batteries” here.