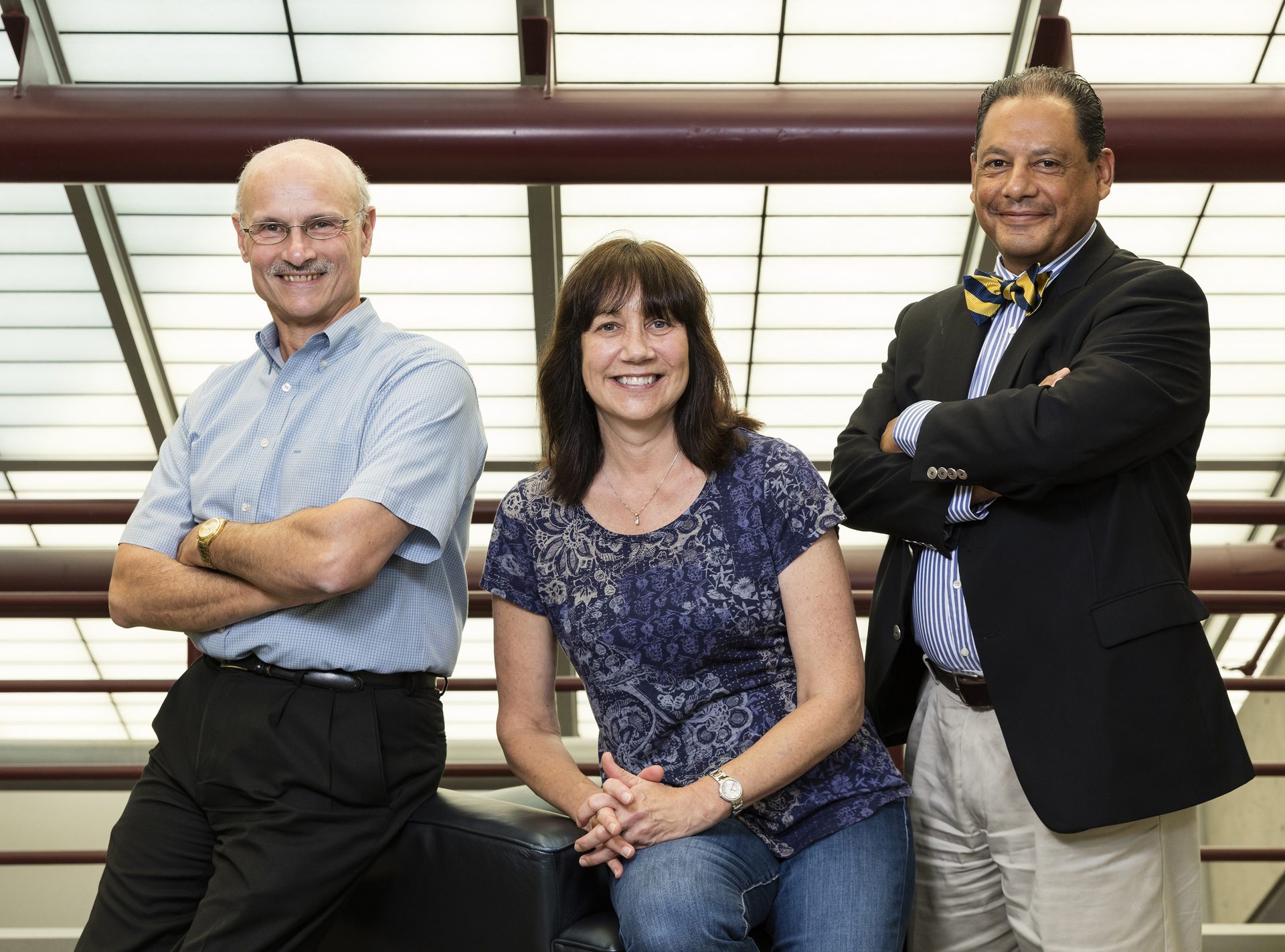
Oak Ridge National Laboratory-led program supported R&D of first widely deployed CMC product
By Dawn Levy, senior science writer at Oak Ridge National Laboratory
This article is an edited and trimmed version from Oak Ridge National Laboratory. It is reprinted with permission. Read the original article. The LEAP engine, introduced in 2016, has more than 25 million aircraft hours of service. The story of the researchers and discoveries that led to its development is still compelling.
Ceramic matrix composite (CMC) materials are made of coated ceramic fibers surrounded by a ceramic matrix. They are tough, lightweight, and capable of withstanding temperatures 300–400 degrees Fahrenheit hotter than metal alloys can endure. If certain components were made with CMCs instead of metal alloys, the turbine engines of aircraft and power plants could operate more efficiently at higher temperatures, combusting fuel more completely, and emitting fewer pollutants.
More than a quarter-century ago, the U.S. Department of Energy began a program, led by DOE’s Oak Ridge National Laboratory (ORNL), to support U.S. development of CMC materials. In 2016, LEAP, a new aircraft engine, became the first widely deployed CMC-containing product. CFM International, a 50/50 joint venture of Safran and GE, manufactures LEAP.
The engine has one CMC component, a turbine shroud lining its hottest zone, so it can operate at up to 2,400°F. The CMC needs less cooling air than nickel-based super-alloys and is part of a suite of technologies that contributes to 15% fuel savings for LEAP over its predecessor, the CFM 56 engine.
“The materials developed in the DOE program became the foundation for the material now going into aircraft engines,” says Krishan Luthra, who led GE Global Research’s development of CMCs for 25 years.
ADVERTISEMENT
GE’s CMC is made of silicon carbide (SiC) ceramic fibers (containing silicon and carbon in equal amounts) coated with a proprietary material containing boron nitride. The coated fibers are shaped into a “preform” that is embedded in SiC containing 10–15% silicon.
ORNL’s Rick Lowden did foundational work in the 1980s that paved the way for DOE programs. The key was coating the ceramic fibers.
“A ceramic matrix composite is different than almost all other composites because the matrix is ceramic and the fiber is ceramic,” Lowden says.
Combining two brittle materials typically yields a brittle material, he says.
But altering the bond between fiber and matrix allows the material to act more like a piece of wood. Cracks do not propagate into the fibers from the matrix around them. The fibers hold the material together and carry the load while slowly pulling from the matrix, adding toughness.
Firing up research on ceramic composites
DOE’s Continuous Fiber Ceramic Composite (CFCC) program ran from 1992 to 2002 and supported industrial development of CMCs by AlliedSignal, Alzeta, Amercom, Babcock and Wilcox, Dow Chemical, Dow Corning, DuPont-Lanxide Composites, GE, and Textron. Its budget averaged $10 million per year, and industry shared costs.
CFCC funded companies to make composites and national labs and universities to characterize the properties of the materials. Efforts were coordinated and funded through ORNL. Lowden wrote the program plan with Scott Richland of DOE and Mike Karnitz of ORNL and co-led support to companies with ORNL’s Karren More, Pete Tortorelli, and Edgar Lara-Curzio and Argonne National Laboratory’s Bill Ellingson.
“We were looking at different fibers and different interfacial coatings and different matrices,” More says of ORNL’s role. “We were involved in understanding the degradation mechanisms and down-selection of the more promising composites and cost-effective techniques for preparing them.
Long before ceramic fibers reinforced ceramic composites, ORNL researchers coated nuclear fuel with carbon and SiC to confine radioactivity inside tristructural-isotropic (TRISO) fuel particles. During experiments in the ’70s, ORNL’s Jack Lackey realized the process could be modified to manufacture ceramic composites more rapidly. With support from DOE’s Fossil Energy Materials Program, his group pioneered a process to do just that.
“You take a fibrous preform, place it in a furnace, and vapor-deposit solids on and around the fibers,” explains Lowden, who was Lackey’s technician. To coat the whole object uniformly, the deposition process must be extremely slow—a half-inch part might take six months to process.
However, the ORNL team found that placing a fibrous mat on a cold plate, heating the top, and forcing gases through the mat sped up the process from months to hours.
“That’s where we got involved in ceramic matrix composites,” Lowden says. ORNL supplied CMCs for years to researchers evaluating CMCs for various applications.
Since CFCC, GE has tested CMCs for more than 2 million hours, including 40,000 hours in industrial gas turbines. Jim Vartuli of GE’s CMC program says DOE support on large industrial gas turbines to get those first demonstrators gave GE confidence that the ceramics could survive high temperatures and stresses in turbines for long periods.
How DOE and its national labs helped industry
CFCC companies brought materials they had made to DOE national laboratories at Argonne for nondestructive evaluation and at Oak Ridge for microstructural characterization and stress and oxidation tests.
“This partnership highlights the value of the national labs,” More says. “We do work that is fundamental and broad to understand materials’ behaviors. We provide necessary information to help the community make decisions about where to go, how to proceed.” New knowledge about how materials degraded helped industry accelerate improvements and optimize manufacturing processes.
Research at ORNL ranged from development by Allen Haynes of environmental barrier coatings that could extend the lives of underlying materials five-fold to nondestructive imaging of materials with thermal cameras by Ralph Dinwiddie.
At Argonne National Laboratory, Bill Ellingson led development of broader nondestructive testing methods to ensure safe continued use of components by monitoring material degradation after intervals of usage. Without damaging the components, the inspections revealed how materials responded in an environment over time.
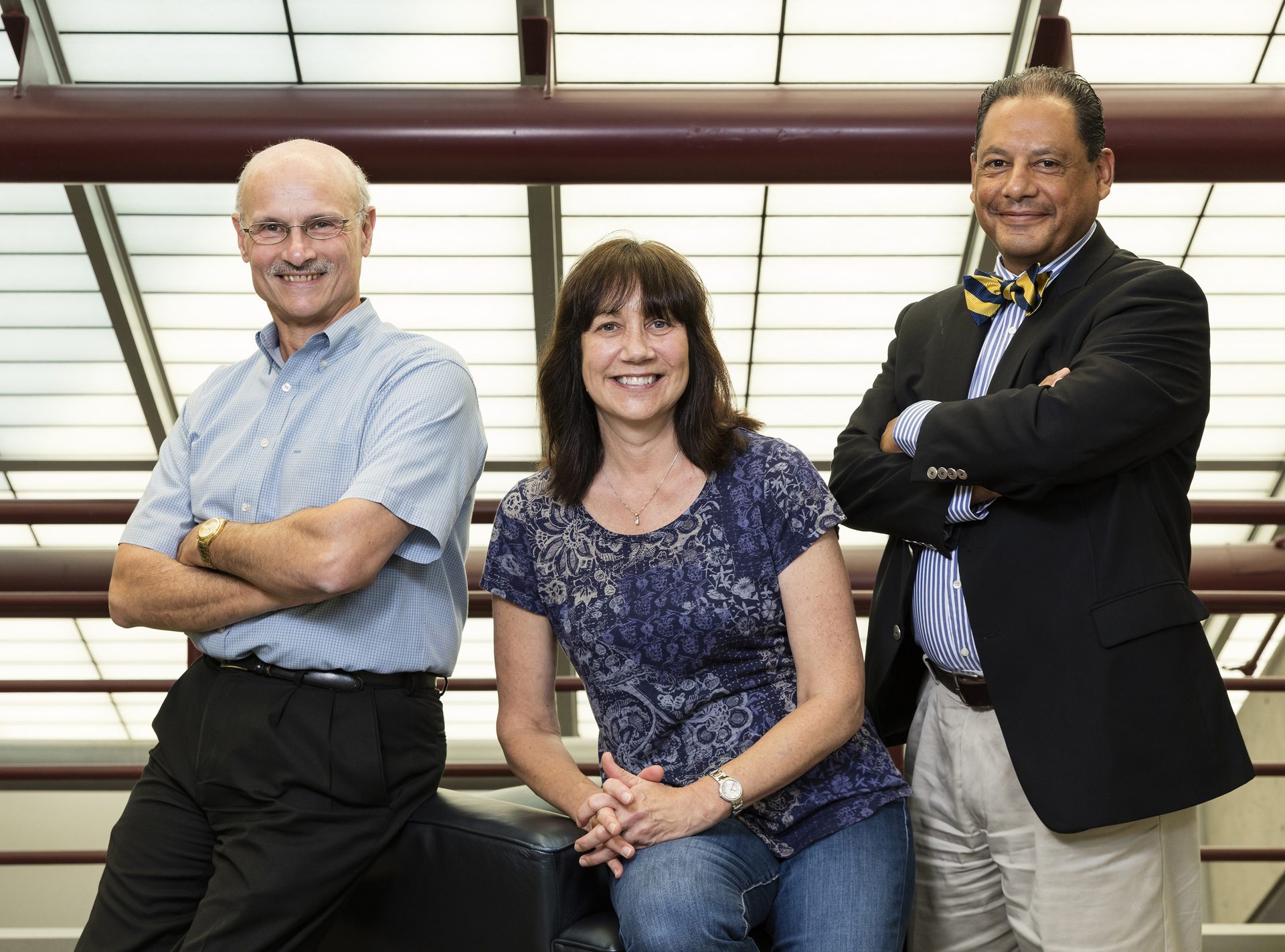
From left, Oak Ridge National Laboratory researchers Pete Tortorelli, Karren More, and Edgar Lara-Curzio were instrumental in advancing CMC technology. Credit: ORNL
With ORNL researchers, Argonne scientists developed several nondestructive inspection technologies that were instrumental in determining component performance.
ORNL’s Pete Tortorelli and H. T. Lin stressed materials in environmental exposure chambers to learn their points of failure. Lab colleagues Jim Keiser and Irv Federer exposed samples to corrosive gases, temperatures up to 2,550°F and pressures up to 500 psi in “Keiser rigs” that simulated conditions in turbines. These methods were also used by More, Tortorelli, and Keiser to screen protective coatings needed in combustion environments.
Meanwhile, More characterized structures of stressed materials.
“Karren More entered the picture as our microscopist, and that changed our world,” Lowden recalls. “To be able to see what was happening with transmission electron microscopy, and understand what was happening at that level, was incredible.”
GE had access to some techniques in-house because of its large infrastructure.
“But we got invaluable help from Karren on the fiber coatings,” Luthra says. “It helped us develop the fiber coatings faster.”
ORNL’s early findings encouraged industry to abandon carbon as a fiber coating. Carbon oxidized, turning into carbon monoxide and carbon dioxide, and volatilized, thinning the coating. ORNL engineers recommended oxidation-resistant boron nitride instead.
Moreover, Edgar Lara-Curzio modeled and tested the mechanical performance of CMC materials under different loading conditions and their resistance to fatigue, creep, and rupture in ORNL’s High Temperature Materials Laboratory. In collaboration with Matt Ferber and Chun-Hway Hsueh, he implemented experimental and analytical methods to characterize the micromechanics of fiber–matrix interfaces.
“These measurements were essential to quantify chemical bonding between fibers and matrix, residual stresses experienced by the fibers, and friction between the fibers and the matrix during fiber sliding,” says Lara-Curzio, noting CMCs are tough mainly because interfacial coatings let fibers slide and bridge matrix cracks.
He and Hsueh provided key information about how a single fiber slides in a ceramic matrix. Lara-Curzio, Ferber, and Lowden then quantified the effect of the thickness of fiber coatings on sliding and discovered a value that optimized mechanical properties. Companies widely adopted this correlation to optimize their composites.
DOE’s Advanced Manufacturing Office (AMO), formerly known as the Industrial Technologies Program, supports applied research, development, and demonstration of new materials, information, and processes that improve U.S. manufacturing’s energy efficiency, as well as platform technologies for manufacturing clean energy products. AMO helped fund this research.
UT-Battelle manages ORNL for DOE’s Office of Science. The single largest supporter of basic research in the physical sciences in the United States, the Office of Science is working to address some of the most pressing challenges of our time. For more information, please visit www.energy.gov/science.